THE HUMAN MOLECULAR AND CARDIOVASCULAR RESPONSES TO GRAVITATIONAL LOADING ON THE LONG-RADIUS vs. SHORT-RADIUS HUMAN CENTRIFUGE
Location: NASA Ames Research Center, 20G Human Centrifuge
Investigators: Howarth, M, Schmidt, MA, et al.
Metabolomics Lead: Schmidt, MA
This study was intended to determine how artificial gravity affects cardiovascular responses during centrifugation. Net fluid (blood) shifts between body segments (thorax, abdomen, upper leg, lower leg) were analyzed to assess the cardiovascular responses to these variables of artificial gravity, as well as to begin to understand potential mechanism(s) underlying the beneficial orthostatic tolerance response resulting from artificial gravity training.
We undertook this pilot study (NASA HRI-246) to determine the practicality of using the NASA Ames 20 G long radius (27.36 ft., 8.8 m) and short radius (8.36 ft., 2.5 m) centrifuge to quantify effects of different G levels, radii, and their resulting gravity gradients on body fluid distribution, cardiovascular regulatory responses, and the effect of lower limb exercise. Subjects were instrumented with four body segment (thorax, abdomen, upper leg, lower leg) bio-electrical impedance (UFI THRIM 2994D) and a high-fidelity 12-lead ECG (CardioSoft; Figure 1). Current injecting electrodes for impedance were placed on the forehead and ankle. The current detecting electrodes were placed near anatomic landmarks for repeatability across subjects and test days.
These were designated the thorax (supraclavicular area to immediately below the pectoral muscle and aligned with the nipple), the abdomen (immediately below he pectoral muscle aligned with the nipple to the iliac crest along the inguinal crease), the upper leg (iliac crest along the inguinal crease to just above the knee), the lower leg (just below the knee to the ankle). High-quality Ambu® Blue Sensor Ag/AgCl electrodes were used for both impedance and the 12-lead ECG.
We also examined two plasma specimens (baseline and endpoint) from three subjects under three separate hypergravity conditions to evaluate experimental design elements related to conducting metabolomics analysis on a very small subject population (Schmidt and Howarth 2007; Howarth 2014). This type of metabolomics pilot was important to conduct in the artificial gravity domain, since there is little metabolomics work applied to artificial gravity. Moreover, it is important to refine the experimental design of such omics studies, given the mathematical challenges of small subject numbers coupled with variable (analyte) numbers in the thousands (and the risk of overfitting the data).
Figure 1. Dr. Mark Howarth monitoring initial measurements from an instrumented subject
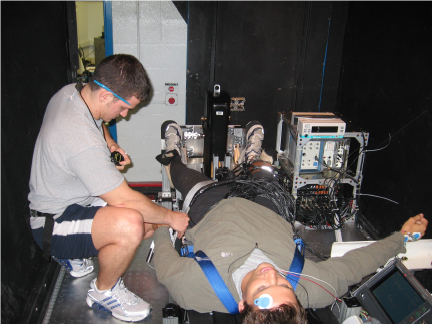
The experimental conditions included:
- Condition A: Short Radius (1–1.25 G) No Exercise (Legs Extended)
- Condition C: Long Radius (1–1.25 G) No Exercise (Legs Extended)
- Condition H: Long Radius (1–2.5 G) Legs Up (Variable Exercise)
The metabolomics portion of the study generated roughly 16,000 molecular features, which were evaluated using principal component analysis. The only condition showing any baseline to endpoint trend was in the three subjects, during the Long Radius-Variable Exercise paradigm, though these results must be taken with caution due to small subject numbers. The study clearly illustrated the unique challenges of small subject and large variable numbers encountered in space-related omics studies, and highlighted the special consideration that must be given to experimental design in such research.
Figure 2. NASA Ames Human Powered Centrifuge (Stenger 2005)
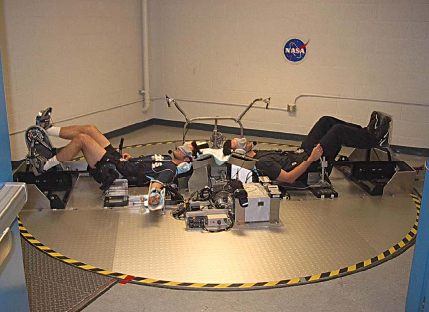
Note: The physiological responses associated with continuous exposure to an alternate gravity source such as Mars (0.38G) are unknown. In fact, human physiological responses to continuous exposure to anything other than 1G are unknown. If it turns out that substantial physiological deconditioning occurs in Mars gravity, then the application of artificial gravity may be required to protect crews during extended stays on the surface of Mars. The only feasible implementation on a planetary surface would be intermittent artificial gravity via a centrifuge (such as the NASA Ames Human Powered Centrifuge; Figure 2). While not expected to be as efficient as continuous artificial gravity from a physiological standpoint, given the gravity gradient effects and intermittent exposure, this procedure may prove effective.
On a centrifuge, artificial gravity is generated by centripetal acceleration. The amplitude of this acceleration varies directly with the radial distance from the center of rotation and with the square of rotational angular velocity. On a short-arm (~ 2 meters) centrifuge, the subject lies on his/her back on radius with the head towards the center and the feet outwards, and the body perpendicular to the rotation axis. In this orientation, the centripetal acceleration is parallel to the body main axis and points toward the head (+Gz, centrifugal force directed head-to-foot). With a short-arm centrifuge, there is a gravity gradient along the length of the body. Because the head is near the center, the GZ level at the head is close to zero; but the GZ can be upwards of two to four G’s at the feet, depending on the rotational angular velocity.